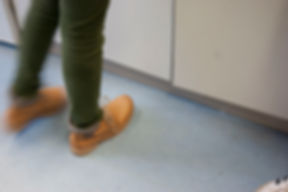
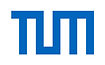



Epithelial branch elongation is a central developmental process during branching morphogenesis in diverse organs. This fundamental growth process into large arborized epithelial networks is accompanied by structural reorganization of the surrounding extracellular matrix (ECM), well beyond its mechanical linear response regime. Here, we report that epithelial ductal elongation within human mammary organoid branches relies on the non-linear and plastic mechanical response of the surrounding collagen. Specifically, we demonstrate that collective back-and-forth motion of cells within the branches generates tension that is strong enough to induce a plastic reorganization of the surrounding collagen network which results in the formation of mechanically stable collagen cages. Such matrix encasing in turn directs further tension generation, branch outgrowth and plastic deformation of the matrix. The identified mechanical tension equilibrium sets a framework to understand how mechanical cues can direct ductal branch elongation.
B. Buchmann, L.K. Engelbrecht, P. Fernandez, F.P. Hutterer, M.K. Raich, C.H. Scheel, A.R. Bausch (2021)
Nature Communications, 12, 2759
Cell-driven plastic remodeling of the extracellular matrix (ECM) is a key regulator driving cell invasion and organoid morphogenesis in 3D. While, mostly, the linear properties are reported, the nonlinear and plastic property of the used matrix is required for these processes to occur. Here, we report on the nonlinear and plastic mechanical properties of networks derived from collagen I, Matrigel, and related hybrid gels and link their mechanical response to the underlying collagen structure. We reveal the predominantly linear behavior of Matrigel over a wide range of strains and contrast this to the highly nonlinear and plastic response of collagen upon mechanical load. We show that the mechanical nonlinear response of collagen can be gradually diminished by enriching the network stepwise with Matrigel. This tunability results from the suppression of collagen polymerization in the presence of Matrigel, resulting in a collagen network structure with significant smaller mesh size and consequent contribution to the mechanical response. Thus, the nonlinear plastic properties and structure of the ECM is not simply the addition of two independent network types but depends on the exact polymerization conditions. The understanding of this interplay is key toward an understanding of the dependencies of cellular interactions with their ECM and sheds light on the nonlinear cell–ECM interaction during organogenesis.
B. Buchmann, P. Fernandez, A.R. Bausch (2021)
The role of nonlinear mechanical properties of biomimetic hydrogels for organoid growth
Biophysics Reviews, 2, 0214041
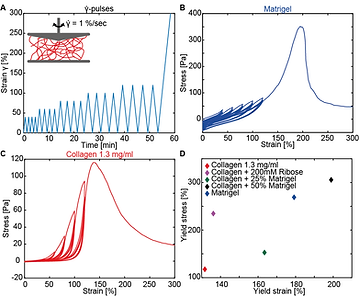

The exceptional mechanical properties of the load-bearing connection of tendon to bone rely on an intricate interplay of its biomolecular composition, microstructure and micromechanics. Here we identify that the Achilles tendon–bone insertion is characterized by an interface region of ∼500 μm with a distinct fibre organization and biomolecular composition. Within this region, we identify a heterogeneous mechanical response by micromechanical testing coupled with multiscale confocal microscopy. This leads to localized strains that can be larger than the remotely applied strain. The subset of fibres that sustain the majority of loading in the interface area changes with the angle of force application. Proteomic analysis detects enrichment of 22 proteins in the interfacial region that are predominantly involved in cartilage and skeletal development as well as proteoglycan metabolism. The presented mechanisms mark a guideline for further biomimetic strategies to rationally design hard–soft interfaces.
L. Rossetti, L. Kuntz, E. Kunold, J. Schock, K. Müller, H. Grabmayr, J. Stolberg-Stolberg, F. Pfeiffer, S. Sieber, R. Burgkart, A.R. Bausch (2017)
The microstructure and micromechanics of the tendon–bone insertion
Nature Materials 16, 664-670
Muscle forces are produced by repeated stereotypical actomyosin units called sarcomeres. Sarcomeres are chained into linear myofibrils spanning the entire muscle fiber. In mammalian body muscles, myofibrils are aligned laterally, resulting in their typical cross-striated morphology. Despite this detailed textbook knowledge about the adult muscle structure, it is still unclear how cross-striated myofibrils are built in vivo. We investigate the morphogenesis of Drosophila abdominal muscles and establish them as an in vivo model for cross-striated muscle development. By performing live imaging, we find that long immature myofibrils lacking a periodic actomyosin pattern are built simultaneously in the entire muscle fiber and then align laterally to give mature cross-striated myofibrils. Interestingly, laser micro-lesion experiments demonstrate that mechanical tension precedes the formation of the immature myofibrils. Moreover, these immature myofibrils do generate spontaneous Ca2+-dependent contractions in vivo, which, when chemically blocked, result in cross-striation defects. Taken together, these results suggest a myofibrillogenesis model in which mechanical tension and spontaneous muscle twitching synchronize the simultaneous self-organization of different sarcomeric protein complexes to build highly regular cross-striated myofibrils spanning the length of large muscle fibers.
M. Weitkunat, M. Lindauer, A.R. Bausch and F. Schnorrer (2017)
Development 144, 1261-1272
